A Raman spectrometer is a device that irradiates a sample with a laser and examines the type and state of a substance from the generated Raman scattered light. Non-contact and nondestructive, molecular structure analysis, crystallinity evaluation, residual stress analysis, etc. are possible.
Configuration of Raman spectroscope
The basic equipment configuration consists of a light source for irradiating the sample with light, a spectrometer that disperses the scattered light, and a detector that detects the scattered light. In addition, with a microscopic Raman spectrometer, by combining a microscope, it is possible to extract information in a minute area of less than 1 μm.
Because the intensity of the Raman scattered light is very weak with respect to the incident light (excitation light), the component performance and optical design cause differences in the device performance.
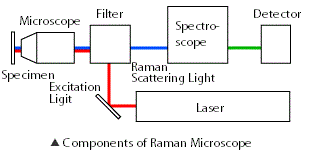
Lightsource
In order to calculate the Raman spectrum based on the wavelength of the irradiation light, it is required that the irradiation light be a stable single wavelength. Furthermore, in order to generate sufficient Raman scattered light, it is necessary to be a high intensity light source. Also, the narrower the line width of the light source, the higher the wavenumber resolution. To meet these requirements, modern Raman spectrometers often use solid-state lasers.
Spectrometer
An important part of analyzing the scattered light produced and examining its contents is generally a polychromator incorporating a diffraction grating. The diffraction grating is grooved, and the finer this number, the higher the spectral resolution. Also, the spectral performance is related to the focal length of the spectrometer, and the longer the distance, the higher the spectral resolution.
Detector
Raman scattered light is so weak that a detector with high sensitivity (quantum efficiency) is required. The detector’s quantum efficiency varies greatly with wavelength, so it is necessary to select the excitation wavelength and measurement wave number range. Today, cooled CCD detectors are used in most cases when using visible light sources.
Microscope
Although the type of microscope is generally upright, depending on the application such as cell observation, it may be combined with the inverted type. Since the laser beam to be emitted is finally focused through the objective lens, it is also important to select an objective lens with a large NA (Numerical Aperture) in order to obtain high spatial resolution.
Variation of specification
How should we choose the device, actually use? It depends on what kind of thing you want to measure, what kind of information you want to extract, and how much accuracy you need. Pursuing one accuracy can lead to trade-offs with other accuracies, as well as significant price differences. Let’s consider each of the components of the basic configuration introduced earlier.
Lightsource
Irradiated light is required to have a stable single wavelength. So what wavelength should I choose? The RAMANtouch selected wavelength is 532 nm as the default. Performance and price are stable by using solid laser. The shorter the wavelength of the light source, the higher the efficiency of Raman scattering and the higher the spatial resolution, but it is more susceptible to fluorescence. When dealing with samples where fluorescence is not a concern and short wavelengths are preferable, 488 nm, 405 nm, etc. may be selected. If you only want to obtain information on the surface of silicon, you may choose an ultraviolet laser that has a short penetration depth into the sample.
On the other hand, at 532 nm, measurement may be difficult due to the influence of fluorescence. At that time, lasers with longer wavelengths, such as 671 nm and 785 nm, are an option. In addition to the problem of fluorescence, there are also fields where many different wavelengths are required, such as carbon nanotube research. Since optical design and optical components differ depending on the wavelength, it is necessary to carefully select which wavelength is the guaranteed performance and whether it is the performance at other wavelengths when multiple wavelengths are mounted on one machine. There is. In addition, when using the near infrared region of 1000 nm or more, it is necessary to combine a special detector accordingly.
Spectrometer
The type of grating in the spectrometer and the focal length are related to performance. A diffraction grating is for separating Raman scattered light generated from a sample for each wavelength, and a thin groove is carved on the surface. Generally, the number of grooves per 1 mm is described as 600 gr (grooves) / mm, 2400 gr / mm, etc. As the number of grooves increases, the wave number resolution increases, but there are also aspects that the wave number range that can be dispersed at one time narrows, and it becomes difficult to detect weak signals. In addition, it is necessary to consider the combination with the wavelength range to be dispersed. The longer the focal length of the spectrometer, the better the spectral performance.
Detector
In most cases, electronically cooled CCD detectors are suitable. There are two types of electron-cooled CCDs, front-illuminated and back-illuminated, and the back-illuminated quantum efficiency is several tens of percent higher than that of the front-illuminated. Also, when using a wavelength of 1000 nm or more as the light source, an InGaAs detector is used. For CCD cameras, detection sensitivity changes according to the quantum efficiency of the wavelength region to be detected. If you purchase a Raman microscope with multiple wavelengths, you also need to pay attention to the quantum efficiency of the CCD you are using.
In addition, some manufacturers have recently proposed EMCCD, which has a short data transfer time. Normally, when measuring a Raman spectrum with a CCD, the actual measurement time is the laser exposure time plus the data transfer time of the CCD. EMCCD is characterized by this short data transfer time, and it can read more spectrum per second than CCD. However, in general, the exposure time required for Raman spectroscopy may take several seconds to tens of seconds, or even several minutes, and the ratio of data transfer time to the total measurement time is extremely small. EMCCDs generally have more noise and weaknesses than CCDs. Therefore, when considering mounting EMCCDs, it is necessary to carefully determine what advantages there are specifically compared to CCDs.
Microscope
A microscopic Raman spectrometer narrows down light to be emitted by a microscope objective lens. The type of microscope is generally upright, but in some fields, such as cell observation, an inverted microscope is combined. The numerical aperture (NA) of the objective lens affects the spatial resolution, so be careful not only with the magnification but also with this NA value. High spatial resolution is also advantageous for detecting minute components in the bulk.
In addition to NA, there are various options for the objective lens depending on the application, an immersion lens that can observe cells in the culture medium such as cells in water, and an oil immersion lens that enhances NA using oil with a high refractive index Besides, there are lenses with a glass correction ring that can observe a sample through a glass plate of around 1 mm.
Stage
The sample stage mounted on the microscope includes a manually operated manual stage, a motorized stage electrically operated using a joystick, etc., and a piezo stage capable of highly accurate scanning. There are two main purposes for stage operation: operation for finding the portion of the sample to be observed, and scanning for Raman imaging. Although a manual stage is convenient for finding the observation site, for example, a motorized stage may be required when selecting the observation site at a fixed interval and proceeding with the measurement automatically.
The scanning accuracy of the motorized stage is usually sufficient for scanning for Raman imaging. According to the Nyquist theorem, in a microscope, if measured at a scanning pitch r / 2 [nm] that is half the spatial resolution r [nm], the original information will be saved (see below). The spatial resolution of the Raman microscope is about 300 nm at the theoretical limit when using a 532 nm laser and a 0.90 NA objective lens, so the scanning accuracy (100 nm) of the motorized stage can be said to be sufficient.
A growing number of manufacturers are adopting a high-precision, high-speed, easy-to-operate laser beam scanning method instead of an electrically driven stage.
Comparison with other analysis methods
As we have seen so far, Raman spectrometers can identify the type of substance, the state of crystals, etc. by analyzing the Raman scattered light generated by irradiating light. So what are the characteristics when compared with other analysis methods?
Comparison with infrared absorption spectroscopy
One of the most commonly compared is the infrared absorption spectrometer(IR). The two are very similar in that they give light to a substance to obtain molecular vibration information, and in fact there is a difference in intensity, but molecular binding information is detected at the same wavenumber. In IR spectroscopy, the activity is high for those with an electric field bias in the molecule, but in Raman spectroscopy there is a difference in that the activity is higher for those in which the bias is induced by an external electric field, complementary Information can be extracted. Infrared absorption spectroscopy is more suitable for detecting highly polar functional groups, but Raman spectroscopy also has the advantage of allowing observation through glass, observation in aqueous solution, and analysis of minute regions.
Comparison with elemental mapping
Some Raman spectrometers can scan a stage or a laser, perform multipoint analysis, and image composition distribution. The spatial resolution is about 1 μm, which is far from electron microscopy, but the phase structure of the polymer blend, which is difficult to identify in elemental mapping or requires special pretreatment, is also identified as a component in the Raman spectrometer. The distribution state can be imaged. In addition, taking advantage of the characteristic of Raman that can be measured through glass or in a state containing a large amount of water, it is attracting attention in fields such as gel-like products and foods that are difficult to measure under vacuum.
Comparison with X-ray diffractometer
One of the typical analyzers to evaluate crystalline substances is X-ray diffractometer. In X-ray diffraction, not only identification of the type of crystal but also the distance of crystal lattice can be grasped by calculation. Raman spectroscopy allows comparison of crystal type and relative crystallinity, but not the inter-lattice distance. Also, it is not practical to measure perfect pure metals. On the other hand, it is possible to obtain the information by targeting the minute area less than 1 μm by squeezing the laser. In addition, it is possible to retrieve information in the area closer to the surface compared to X-rays. Detailed information as bulk is X-ray diffraction, and Raman spectroscopy is suitable for extracting surface information and minute distribution information.
In addition, it is an analyzer that can be relatively easily expanded in function, for example, by setting reaction fields such as a heating stage on a stage and tracking changes over time, because it can be measured with ease in microscopic observation in a normal environment. It can be said.